Previous posts: I, II, III, IV, V, VI, VII, VIII
Welcome, one and all, to your series of posts on magnetism. This marks the ninth instalment, and it is with great pleasure that I announce we will be covering perhaps one of the richest, and most interesting magnetic phenomena today; namely, ferromagnetism. As somewhat of a test, I won't be bothering you with equations; rather, we will take a more qualitative approach. If this troubles you, please do let me know by publishing a comment. Lets get started.
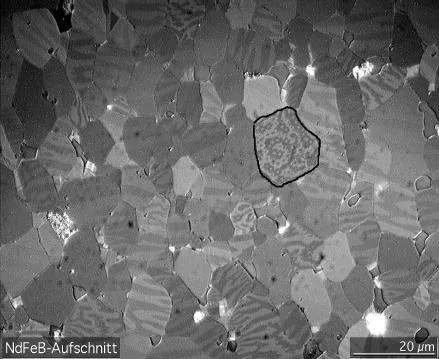
'Microcrystalline grains within a piece of NdFeB (the alloy used in neodymium magnets) with magnetic domains made visible with a Kerr microscope. The domains are the light and dark stripes visible within each grain.'
A ferromagnet has a spontaneous magnetisation, even in the absence of an applied field. All the magnetic moments lie along a single, unique direction. This effect is generally due to exchange interactions, which arise as a consequence of the Pauli exclusion principle, and lie at the heart of long range magnetic order.
There are a number of different types of exchange interaction; namely, direct exchange, indirect exchange, superexchange (which manifests as indirect exchange in ionic solids), double exchange, anisotropic exchange, the list goes on. The crux of the matter, however, boils down to electrostatic interactions arising because charges of the same parity cost energy when they are close together, and save energy when they are apart.
As alluded to previously, this is a consequence of the fundamental principle of Pauli, which demands that no two fermions (spin-1/2 particles, such as electrons) can share the same quantum state. For electrons, the overall wavefunction must be antisymmetric.
It is indeed surprising, and perhaps even amusing, that we need to think about such deep concepts in quantum mechanics, when the reality of the situation we seek to explain is characterised by an inconspicuous bar magnet and a smattering of iron filings on the desk!
We have established that at zero applied field, we have some non-zero, net magnetisation. What this means, is that there exists some ordering of the spin states within the ferromanget - they are lining up, relative to eachother. Now, what happens when we subject our ferromagnet to a magnetic field? As you might expect, the degree of alignment increases, until all the spin states are aligned, and we describe the magnetisation as being saturated - they can't be any more aligned than in this configuration.
We should be very careful, though in making such statements, because we are yet to consider the effect of temperature. All that we have said is valid below the so-called Curie temperature. This is the temperature at which a phase transition takes place. Below the Curie temperature, we have spontaneous magnetisation, and aligning of spins when a field is applied, but at temperatures exceeding this critical point, the thermal energy is sufficient to destroy the long range order, resulting in no net magnetisation.
There's another, somewhat subtle, phenomenon that occurs within ferromagnets, that we have not yet discussed, and is the formation of domains.
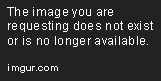
It turns out, that it is energetically favourable for neighbourhoods of spin states to emerge, wherein all the magnetic moments are aligned relative to one another. The formation of domains minimises magnetostatic energy. You can get situations in which adjacent domains point in opposite directions, as is the case in antiferromagnetism, leading to an overall magnetisation of zero, but in the case of pure ferromagnetism, an applied field brings about long range alignment of spin domains. We mention, in passing, that between adjacent domains there exists a boundary called a domain wall, separating domains of perpendicular magnetisation.
I made a post, yesterday linking to a video of a talk given by Edward Witten on emergent phenomena in condensed matter (which I urge you all to watch), pointing out that ferromagnetism can be classified as such.
If we suppose that we have a ferromagnetic sample and the local temperature is above the Curie temperature, then there will be no long range magnetic order - all the spin domains will be misaligned, and jumbled up, yielding no net magnetisation. Now, lets suppose that we cool the sample below the Curie temperature. Something remarkable happens, which we have essentially described above, which is called spontaneous symmetry breaking. The highly symmetrical disordered state gains long range order as the spin domains become aligned. A phase transition has taken place, and the overall symmetry of the system has been reduced.
Thank you for reading.
References & Further Reading:
- Magnetism in Condensed Matter, Stephen Blundell, Oxford University Press, New York, 2003.
- Physics of Magnetism, Sōshin Chikazumi, Wiley, 1964.